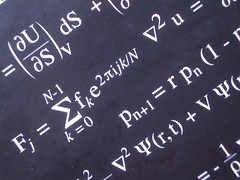 |
The title of this post comes from Dylan Thomas, And Death Shall Have No Dominion (1933). Here I give a non-technical essay on the interplay between geometry and physics, which I hope with give some of the readers a better idea of why I do what I do. Please enjoy and leave feedback if you like. |
Geometry and physics: Though lovers be lost love shall not
To paraphrase a certain Polish mathematician: “the most important ideas in mathematics come from physics”. While there is no reason why mathematics — as mathematics — should come from physics, there is some deep connection between mathematics and our understanding of the Universe. Wigner in 1960 in his famous “The Unreasonable Effectiveness of Mathematics in the Natural Sciences” article, noticed how the mathematical structure of physical theories can lead to new physical insight. And of course, physical insight can lead to new mathematics. By physics, I will mean the construction of mathematical models of natural phenomena and the comparing of the predictions of these models against nature.
From the very nature of physics it is clear that there is at least some superficial relation with mathematics. After all, physics uses mathematics. However, physics is not mathematics in the sense that mathematical constructions in physics should have (maybe not directly) some meaning. There must be some relation of the mathematics to a physical law. In mathematics, there is no such constraint that any of it have any meaning beyond what it mathematically means. It is a complete mystery as to why nature seems generally amenable to being understood in terms of abstract mathematics.
The deep interconnection between mathematics and physics seems especially true when focusing in on geometry: literally geometry means `Earth measurement’. At the most basic level, geometry is the study of spaces, which are understood as collections of points, together with a notion of points being `close to each other or not’, and usually with some further mathematical structures on them, such as a notion of the distance between to near by points. But this is definition in terms of points is not enough to cover the modern usage of `geometry’. So, what is geometry and where does it come from? Moreover, what has the study of spaces got to do with physics?
The first work on synthetic geometry is the book Elements written Euclid of Alexandria (c.325–265 BC). In this book an axiomatic approach to plane geometry, so parallel lines on flat surfaces etc., is established. For example, the internal angles of a triangle on the plane always add up to 180 degrees. However, curves, circles and spheres had been known about since antiquity. Solid geometry — the study of three dimensional objects — was needed as soon as humans started to imagine buildings such as domes and pyramids. In addition to this, the heavenly sky can be imagined as the inner surface of a dome speckled with stars — at least as we see it, and ancient astronomers saw it!
Methods of calculating the volume of simple regular three dimensional objects were developed. For example, the ancient Egyptians knew how to calculate the volume of pyramids and chambers therein: they were the mummy of all modern mathematicians! Archimedes (287–212 BC) in his `eureka’ moment realised that one could deduce the volume of three dimensional irregular objects based on the amount of water they displaced. However, Archimedes was unable to actually calculate volumes in any generality.
In another direction, Apollonius of Perga (c.262–190 BC) showed that the regular curves — circles; ellipses; parabola; and hyperbola — can be formed by cutting the cone, hence conic sections. Amazingly, in Newtonian gravity (circa 1686) the orbits of the two massive bodies are described by conic sections. This is part of the unifying power of mathematics: the mathematics involved in cutting cones is exactly the mathematics needed to describe orbits, for example the path of the Moon around the Earth! These mathematical coincidences are abound.
The most important mathematical works on conic sections — as far as our story goes — are that of Descartes (1596–1650) and Fermat (1601–1665), who in the 17th century brought algebra in to the game. Conic sections can be described by algebraic equations via coordinates — analytic and algebraic geometry were born! \par
The use coordinates (eg. x and x on the plane) opens up the use of calculus in geometry. Newton’s differential and integral calculus allows for methods of calculating gradients of curves, areas under curves, the volumes of objects etc. — calculus today is a common method of torturing undergraduate students! Differential geometry was born … or at least the seeds of the theory were planted by Newton (1642–1727) and Leibniz (1646–1716). One should not forget that much of Newton’s inspiration in developing calculus comes from his work on classical mechanics: so the mathematical description of the motion of massive bodies.
Curved surfaces – such as the sphere – represent non-Euclidean geometries. Lines drawn on them violate the axioms of Euclid’s plane geometry: this was seen as a real problem by mathematicians. It was Eugenio Beltrami (1835–1899) who showed that hyperbolic geometry is consistent: this is the geometry of surfaces of constant curvature for which the internal angles of a triangle add up to less that 180 degrees. Similar results were obtained for spherical geometries, so geometries of constant curvature for which the internal angles of triangles add up to more that 180 degrees.
Bernhard Riemann (1826–1866) in his PhD thesis extended the work of Beltrami to surfaces that have non-uniform curvature, and to higher dimensions. The work of Riemann allowed algebra and calculus to be applied to spaces known as smooth manifolds, i.e., spaces such that every `small piece’ of them looks like a `small piece’ of the n-dimensional plane for some integer n. One should keep in mind the relation between a globe and a map: any small piece of the globe can be represented on a sheet of paper as a map, and points on the globe are then represented by two numbers, the coordinates with respect to the given map. The notion of a smooth manifold underpins Einstein’s special and general relativity, as well as Maxwell’s theory of electromagnetism, Yang–Mills theories and classical mechanics: even thermodynamics has a geometric formulation!
It is worth saying a little more about Einstein’s general relativity (1916). This theory is a theory of gravity, and to date it is the most accurate theory of gravity we have. Moreover four dimensional smooth manifolds are central to the theory. Einstein took the earlier idea that space and time should be unified into space-time seriously, we have one time coordinate and three space coordinates. Einstein then told us that gravity is not your typical force, but rather it really is due to the local shape of space-time! The mathematical theory of curved smooth manifolds is vital to our understanding of gravity and the Universe as a whole, and vice versa, physics has been the impetus for many mathematical works on curved smooth manifolds.
There is a duality between a space and the algebra of functions on that space ( i.e., maps from that space to the real or complex numbers). Loosely, if you know the algebra of functions on a space, then you know the space. The algebra of functions on a classical space is commutative: the order of pointwise multiplication does not matter. We can imagine a more general notion of a `space’ by considering any algebra — not necessarily commutative — as the algebra of functions on some `space’. The phase space of quantum mechanics, that is the `space’ of positions and momenta of a quantum particle, is a noncommutative geometry.
A quantum theory of gravity could be some kind of noncommutative geometry: both string theory and loop quantum gravity suggest noncommutativity of space-time at some level — both the loopers and p-braners agree on this! Trying to make sense of physics at the smallest scales pushes what we mean by geometry well beyond our original understanding. Noncommutative geometries are in general not set theoretical objects, i.e., they do not consist of a collection of points — it is all rather pointless!
There is a kind of `halfway house’ between classical and quantum geometry: here I refer to supermanifolds as defined by Berezin and Leites in 1976. Without details and being very loose, a supermanifold is a `manifold-like object’ which comes with some coordinates that commute with all the coordinates, and some coordinates that anticommute amongst themselves. By anticommute we mean that they pick up a minus sign when we exchange the order they appear in expressions. In particular we have some coordinates that square to zero!
Supermanifolds play the role of manifolds when, for example, fermions such as the electron are present in the theory. If we want to develop a `classical’ theory of fermions then we must employ objects that anticommute: one can justify this using the Pauli exclusion principle — no more than one fermion can be in a given quantum state, while for bosons there is no such restriction. Heuristically, one can say that bosons like to be together, while fermions are rather more like hermits.
Supermanifolds offer a conceptual and geometric way to treat bosons and fermions on equal footing: supermanifolds are the geometry of supersymmetry. In short, supersymmetry is an operation that allows us to `rotate’ a boson into a fermion and vice versa. It turns out that this is not just a neat way of unifying bosons and fermions, but theories that posses supersymmetry can have remarkable mathematical and phenomenological properties — we await CERN’s confirmation that nature uses supersymmetry!
Another amazing link between geometry and physics can be found in mirror symmetry which relates pairs of particular manifolds called Calabi-Yau manifolds. Superstring theory is 10 dimensional, yet our physical world appears 4 dimensional — one time and three space dimensions. To overcome this discrepancy one can postulate that 6 of these dimensions is `scrunched up tightly’, and all we see is four dimensions on all but the very smallest scales. These compactifications as they are known, are Calabi-Yau manifolds, and different compactifications in general lead to different physics. However, it was noticed in the late 1980s by Dixon, Lerche, Vafa, and Warner that two different versions of superstring theory (type IIA and IIB) can be compactified on two different Calabi-Yau manifolds, yet lead to the same physics. In this case the two Calabi-Yau manifolds are said to be mirror duals, and the symmetry between the physics is known as mirror symmetry. This pairing of Calabi-Yau manifolds is now an active area of mathematical research with much effort devoted to carefully understanding the intuitive physics based picture.
In conclusion, not only has geometry been essential in developing physical theories, but these theories then push our understanding of geometry and lead to new mathematics. I have only touched upon a tiny part of this interrelation. There are a great number of other things I could have described and new links are being uncovered all the time. What will future mathematicians understand by the term `geometry’ is anyone’s guess. However, I am sure it will be closely related to our understanding of the physical Universe.